Batteries & Fuel Cells
Powering the Vehicles of the Future
We are working to build batteries and fuel cells that are safer, more powerful, cheaper, and longer-lasting. Our collaborators include many of the U.S. national laboratories as well as leading universities and industry partners.
Batteries & Fuel Cells
Carbon-Free Transportation Technologies
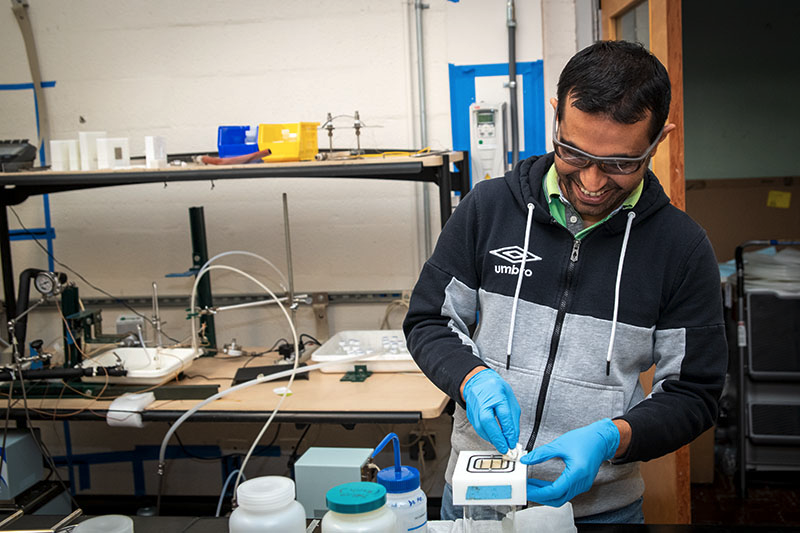
Our researchers focus on resolving the critical roadblocks to enable transportation with high-energy batteries, hydrogen fuel cells, and electrochemical and photo-electrochemical methods for fuel generation. We bring deep expertise in thermo-electric laser technologies, electrochemistry, materials science, characterization, and theory to solve the challenges in these systems.
Next-Generation Batteries
Low-emissions transportation technologies such as plug-in hybrid and all-electric battery vehicles require next-generation batteries featuring safety, high energy density, long life, and low cost.
Scientists at Lawrence Berkeley National Laboratory (Berkeley Lab) and University of California, Berkeley, conduct innovative research to understand the basic science and overcome technological barriers to next-generation batteries. Rechargeable battery technology improvements are limited by the costs of component materials and the fundamental chemical and mechanical instabilities that impede vehicle battery development.
Berkeley Lab’s Energy Storage Group devotes substantial effort to lithium-ion (Li-ion) batteries, which are promising for transportation applications, and the Group is developing batteries for electricity grid-scale applications such as flow batteries. Lab scientists are improving lithium battery performance while also developing new battery chemistries using materials that are more common, less expensive to produce, and more friendly to the environment such as sodium and zinc.
Next-Generation Fuel Cells and Fuel Generation
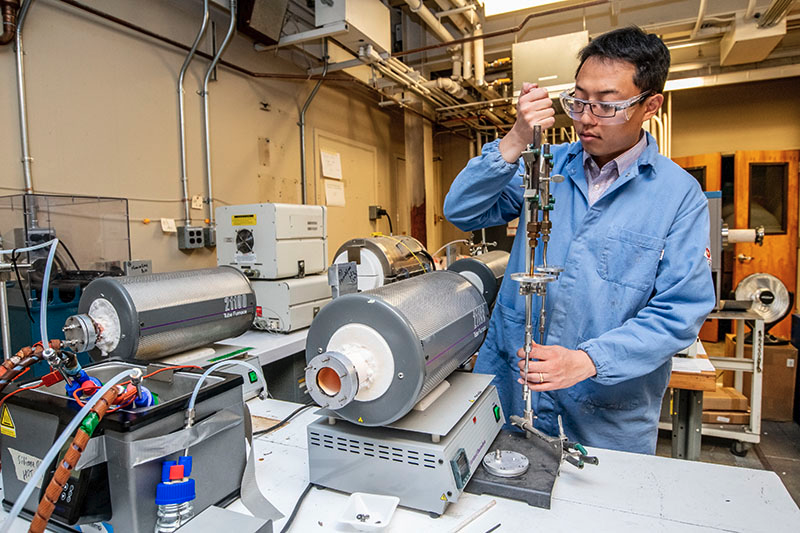
Research in electrochemical energy-conversion devices continues to expand due to their potential to provide clean, renewable, and sustainable energy technologies for transportation applications. The Energy Conversion Group focuses on finding ways to make hydrogen fuel cells and electrochemical and photo-electrochemical fuel generation a viable solution for the transportation sector. They research fuel cell performance and durability using multiscale mathematical modeling and associated advanced diagnostics. They approach these challenges by understanding and optimizing next-generation fuel-cell and related energy-conversion components and materials— mainly through physics-based, multiscale modeling of cell behavior and advanced diagnostics of cell properties and phenomena.
The work focuses on the exploration of transport phenomena, including charged and neutral species, and structure/function/property relationships of the essential components common to many of these technologies to improve device performance and durability. The core team includes electrochemists, chemical engineers, mechanical engineers, theorists, material scientists, and organic chemists.
The Lab’s Facilities, Data, and Tools
Berkeley Lab’s Materials Project provides these scientists with datasets and tools for designing new materials for energy storage and energy conversion devices.
The National Energy Research Scientific Computing Center provides computational resources and expertise to accelerate materials discovery.
Berkeley Lab is also home to some of the most powerful experimental resources in the world, from synchrotron light sources and spectrometers to powerful microscopes. This combination of scientists, tools, and facilities has created one of the world’s leading centers for advanced battery and fuel cell research.
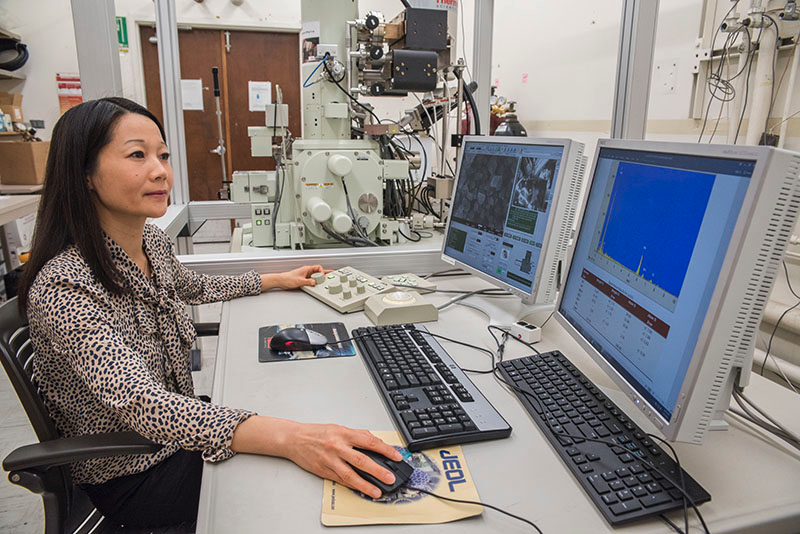
Collaborations and Partnerships
Data, Tools, and Facilities
Our Team is made up of a diverse group of researchers from Berkeley Lab, the University of California, and members of other national laboratories, industry partners, and academia.